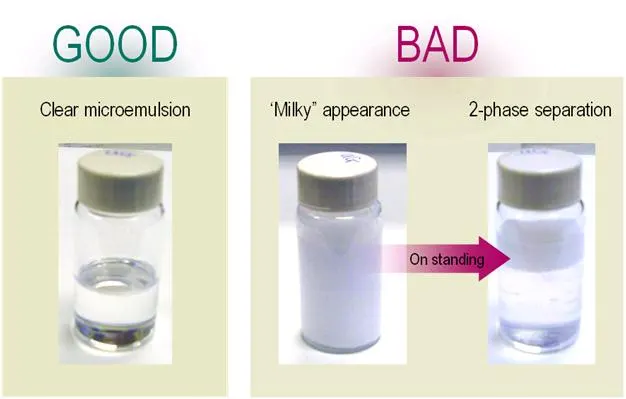
Overview
Preparation will vary by sample type. Depending on the procedure, specific liquid scintillation cocktails may need to be used, and the choice of cocktail will impact the efficiency and the results. The goal is to obtain a liquid sample prior to counting in order to get a better mixture with the scintillation cocktail, and to assure that maximum radiation will reach the scintillator molecules.
Good sample preparation is the art of Liquid Scintillation Counting. A variety of sample types of different origin require a selection of LSC cocktails to finally obtain the best counting results. Homogeneity of sample and cocktail is essential to assure the optimum result for radioisotope detection by LSC.
The pictures below show the proper sample appearance to be clear and homogeneous, milky samples will at the end result in a 2-phase separation.
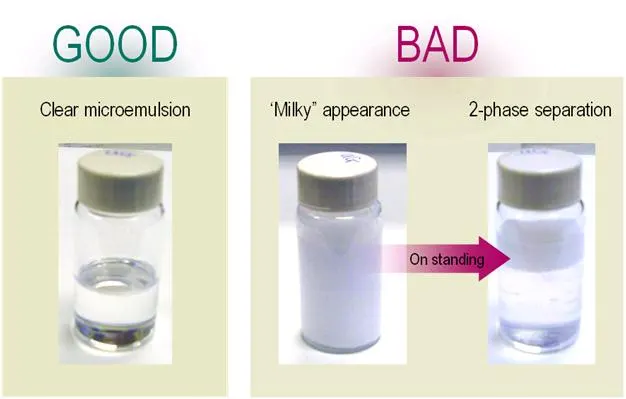
Sample preparation
A poorly prepared sample can only produce bad or unreliable results, regardless of how good the Scintillation Counter is.
Some of the common sample categories are described below, and many application notes have been published for various sample types. You can also contact Revvity Technical Support for further help and advice.
Liquid samples
Aqueous solutions
Aqueous samples are the most common type of sample encountered in LSC, simply because most radioactive species are aqueous-soluble. The aqueous solution may be a buffer or just water. Typical buffers include Tris, chlorides, phosphates, PBS (phosphate buffered saline) and even solutions of sucrose in water.
Buffered solutions
These samples can be further categorized by the ionic strength of the aqueous solution.
Inorganic Anions (Chlorides, Sulfates, Phosphates, Nitrates, etc)
Di- and tri- valent anions sometimes cause instability. The effect on counting results will be both volume- and concentration-dependent. In addition, some metallic salts form colored solutions, which can lead to quenching.
- Recommendations: 1) Select a cocktail suitable for use with these difficult anions with resistance to color quenching Ultima Gold™ and HiSafe™ series); 2) dilute sample with water or increase cocktail volume
Acidic solutions (Hydrochloric Acid, Nitric Acid, Sulfuric Acid, Phosphoric Acid, TCA, Acetic Acid etc.)
Certain strong mineral acids interact with the cocktail components causing color development and performance changes. Concentrated sulfuric acid will cause color formation by sulfonation of the cocktail. TCA can result in acid-induced luminescence.
- Recommendations: 1) Do not add concentrated mineral acids to the cocktail; 2) always dilute the acid with water; 3) use a cocktail which has proven performance with acidic samples (Ultima Gold AB or Ultima Gold LLT)
Alkaline solutions (Sodium Hydroxide, Potassium Hydroxide, etc.)
Alkaline samples often cause chemiluminescence in LSC cocktails. Sometimes color formation occurs.
- Recommendations: 1) Use a cocktail with proven resistance to chemiluminescence (Hionic-Fluor™ and Ultima Gold™); 2) dilute the alkali to reduce the luminescent effect; 3) acidify the cocktail / sample mixture; 4) do not store alkaline samples for days; 5) introduce a waiting period of approx. one hour before starting alkaline sample counting, when chemiluminescence occurs.
Organic solutions
Functional groups present in organic solvents might have a quench effect on a counting assay. This chemical quenching effect depends on the electronic interactions inherent to the group type, and decreases from left to right:
Nitro > Sulphide > Halide > Amine > Ketone > Aldehyde > Organic Acid > Ester > (Water) > Alcohol > Ether > Hydrocarbon,
- Recommendations: 1) Change the organic solvent to one with less quenching (e.g., if possible prefer dichloromethane to chloroform); 2) use a more chemical quench-resistant cocktail like the Ultima Gold family; 3) always check to ensure that the cocktail is accepted into the mixture
Water/Organic solvent mixtures
Mixtures of water with organic solvents behave unexpectedly (e.g. the cocktail may have a high loading capacity for water and high loading capacity for methanol, but the capacity with a 1:1 mixture of water:methanol might be very low).
- Recommendation: Change to a different cocktail (e.g., flow cocktails that are designed for use with such water/organic solvent mixtures: the safer Ultima Flo™ family, OptiScint™ Flow or the classical Flo-Scint™ family)
Biological samples
Biological samples are very commonly analyzed in labs performing environmental studies or studying drug metabolism (ADME-Tox). Typical samples include blood, plasma, serum, urine, feces, homogenates, ground tissue, bacteria, and cells. Depending on the sample type, the biological material can either be directly mixed to the cocktail with no or little pretreatment, or a treatment/solubilization may be needed prior to scintillation cocktail addition.
Counting by direct addition
Direct addition of sample to cocktail is only possible with liquid samples containing little proteinaceous material. Typically, the direct addition of raw sample to a suitable cocktail can be performed when working with plasma, serum, cerebrospinal fluid (CSF), or diluted urine.
Plasma and serum
Up to 1 mL of plasma or serum can be added to certain cocktails and allow reproducible counting. For this method, Ultima Gold is recommended. You might also try adding up to 1 mL of either ethanol or isopropanol per 10 mL cocktail, to suppress the appearance of protein precipitates. With difficult samples, such as plasma and serum from smaller animals, it may not be possible to completely suppress the appearance of the protein precipitates. In this case, the only solution is to complete counting within 24 hours of sample preparation.
Urine
Cocktail selection dictates the sample loading for urine samples, but sample volume is generally restricted to less than 3 mL to minimize the effect of color quench. Ultima Gold (up to 8 mL in 10 mL cocktail) and Ultima Gold LLT (up to 10 mL in 10 mL cocktail) are recommended. With these cocktails, it is possible to add up to 1 mL of either ethanol or isopropanol per 10 mL cocktail to suppress the appearance of the protein precipitate. When it is not possible to completely suppress the appearance of protein precipitates, the only solution is to complete counting within 24 hours of sample preparation. With difficult samples, such as urine from smaller animals, there may be significant problems with sample/cocktail incompatibility. In this case, it may be necessary to either keep the urine sample volume below 0.5 mL per 10 mL cocktail or to dilute the urine sample with an equal volume of deionized water.
Counting after solubilization
Application Note on Sample Solubilization
Solubilization describes the action of chemical reagents on the chemical bonds of a macromolecular structure to affect a structural breakdown (or digestion) into smaller, simpler subunits where after the total preparation can be dissolved directly in a LSC cocktail. The solubilization process usually involves heating the sample/solubilizer mixture at elevated temperature (40° to 65°C) for periods ranging from <1 to 24 hours, until a homogeneous mixture is formed. Certain samples that remain colored after solubilization are optionally treated with hydrogen peroxide.
Chemical solubilizing reagents used should be capable of rapid and complete digestion with respect to both small and large sample sizes. Fundamentally, the major classifications of solubilizing agents are:
Alkaline systems
The mode of action of “alkaline systems” is solubilization by hydrolysis of peptide bonds and a wide range of samples including animal tissues, blood, urine, bone tissue, muscle, amino acids, nucleic acids and proteins can be digested with these reagents. Revvity offers three alkaline solubilizers:
- Soluene-350™ is a strong organic base, formulated with toluene, that has an excellent capacity for the solubilization of wet tissue, aqueous tissue homogenates, proteins, nucleotides and plant material
- SOLVABLE™ is an aqueous-based solubilizer that has an excellent capacity for the solubilization of wet tissue, aqueous tissue homogenates, proteins, and nucleotides. It can thus replace Soluene-350 for many applications, excluding plant material, and increases safety in the laboratory due to its aqueous nature
- Hyamine Hydroxide 10-X is a quaternary ammonium hydroxide solution. It can be used to solubilize many biological tissues and as a 14CO2 trapping agent. Solubilizes many biological tissues; resistant to chemiluminescence. Recommended 14CO2 trapping agent for helicobacter pylori and urea “breath” test studies
Acidic systems
The mode of action of “acidic systems” is sample solubilization by the action of certain strong acids, usually including oxidizing properties. Samples such as cartilage, bone, collagen fibers, and dried and hard plant samples can be digested by these reagents. Occasionally mixed acid reagents, and acids with an added oxidizing agent, are preferred due to their increased oxidative power.
Sodium hypochlorite
A special useful reagent is sodium hypochlorite, whose mode of action is alkaline, oxidative bleaching. This is particularly useful when dealing with plant samples, especially those containing chlorophyll, where the sodium hypochlorite effectively prevents color quench in subsequent liquid scintillation counting by bleaching out all the color present.
Counting after oxidation
Sample oxidation consists of the combustion of the sample in an oxygen-rich atmosphere. Oxidized tritium takes the form of tritiated water (3H2O) and is collected as water, oxidized 14C is collected in the form of carbon dioxide (14CO2).
A comparison between solubilization and oxidation shows that solubilization contains more steps and is consequently more labor-intensive, while the oxidation method requires specific instrumentation, the sample oxidizer.
The decision of whether to use solubilization as the preferred method of sample preparation depends primarily on the nature of the sample and the number of samples that need to be processed. When the number of samples is low, then solubilization is usually the method of choice. Because sample combustion is suitable for any organic and even some inorganic samples, the selection of this method is usually governed by the number of samples that need to be processed. When the sample load exceeds 50 per day, then sample oxidation becomes the method of choice for many sample types.
Sample oxidation offers the advantage of eliminating any color formation or chemiluminescent background from the sample. In addition, it is a unique method to physically separate tritium and 14C in case of dual-labeled samples. The counting of both isotopes will then be performed separately, and no specific software will be needed to calculate the contribution of one isotope versus the other in the total counting results of the sample.
Once the sample is oxidized, two specific scintillation cocktails are used to count the oxidation products:
- Monophase™ S is a pseudocumene-based cocktail specifically formulated for counting pure water samples collecting the tritiated fraction of oxidized samples
- Permafluor™ E+ is a pseudocumene-based cocktail specifically formulated for counting 14CO2 samples that are trapped in Carbo-Sorb E
Solid support
Filters
Filter counting/ solid support counting should be considered as heterogeneous counting. The main difference between heterogeneous counting and the classical liquid scintillation homogeneous counting is that heterogeneous counting relies on 2 pi geometry, while 4 pi geometry applies to homogeneous counting.
In homogeneous counting (vial on the right, below), the sample is completely "dissolved" in the liquid scintillation cocktail. Therefore, the photons of light, which are emitted as the end result of the energy transfer process within the liquid scintillation cocktail, are free to radiate in any direction.
In heterogeneous counting (vial on the left, below), this freedom is restricted by the presence of the filter or membrane which absorbs both the kinetic energy of beta-particles and the photons of light passing in one plane. Therefore, the emitted light can only occupy the surface area of a hemisphere.
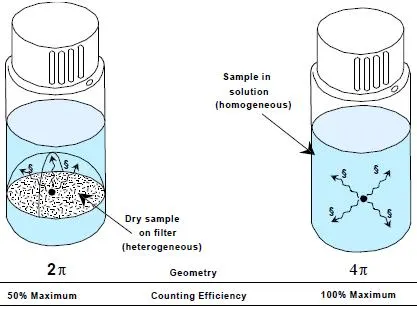
Filter counting
The relatively simple filter counting technique entails isolating or collecting the sample (DNA, TCA precipitates, tritiated thymidine, etc.) on a solid support (glass fiber filter, filter paper, membrane, etc.), drying it (typically), then placing in a scintillation vial with a small volume of an appropriate scintillation cocktail for counting (after ensuring that the filter is completely wet).
In practice, there are several filter types that can be used to isolate or collect various sample types for LSC analysis. The choice of filter type will depend upon both the nature and particle size of the sample, however glass-fiber filters are recommended if at all practical. The difficulty in counting on solid supports is that when the sample is immersed in a cocktail, several situations may develop:
- Partial elution: The sample may be partially eluted by the cocktail. The soluble fraction is counted with 4 pi geometry, whereas the insoluble (filter bound) portion is counted with 2 pi geometry. This makes the measurement under these circumstances irreproducible, unless an equilibrium is reached over time (checked by repeating counting of the sample, over several hours, with a constant count rate being obtained over time). If no equilibrium can be reached accurately and repeatedly, then it is highly recommended to change the assay parameters to convert the system to a complete elution situation.
- No elution: The sample remains bound to the solid support. The efficiency and reproducibility of counting will depend on the magnitude of the beta-energy, the nature of the filter or solid support, its orientation in the vial, and the size of the sample molecule. Sample quench is constant and "simple CPM mode" on the liquid scintillation counter is preferred as external standard quench correction cannot be employed. A simple method to confirm that a no elution situation exists is to decant the cocktail into another vial and recount the cocktail - absence of activity confirms that no elution has occurred and that the sample is completely bound to the filter.
- Complete elution: The sample is completely dissolved/eluted in the cocktail. A true homogeneous state is obtained, and the counting is performed as in a classical liquid scintillation counting assay.
The choice of cocktail depends on several parameters:
- If the filter is dry, a lipophilic cocktail can be used. If not, counting requires an emulsifying cocktail.
- If the filter material is made of cellulose nitrate, mixed cellulose esters or PVC, it can be dissolved using Filter-Count cocktail. When using cellulose acetate filter materials, the recommended reagents for trouble-free counting are Soluene-350 (to completely dissolve this filter type) followed by addition of Hionic-Fluor™ scintillation cocktail. For other insoluble filter types, you will need to check whether the sample can be eluted from the filter.
- The cocktail should be suitable for the instrument on which the filter will be counted. Some scintillation cocktails are specifically formulated to reach the highest counting efficiency on certain counters.
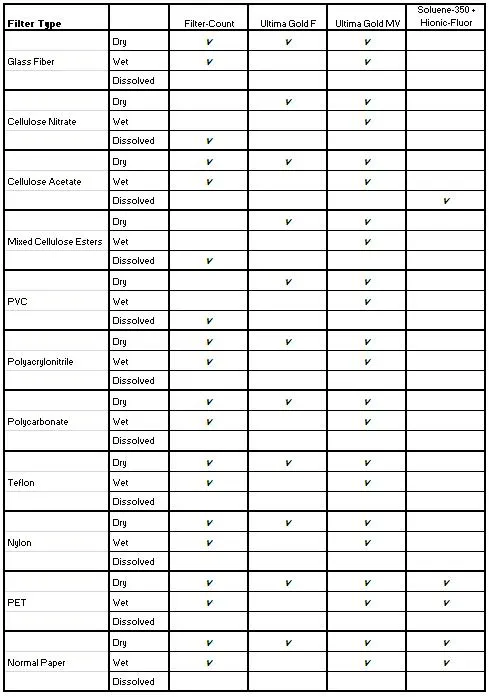
Filter table
Swipe assays/smear tests
An extremely common application using filter counting routinely performed in many radioactivity-authorized laboratories is the swipe assay, often referred to as "smear" or "wipe" tests. Typically, swipes are performed to monitor the presence of removable surface contamination from low energy beta−emitting radionuclides such as 3H, 14C and 35S. Swipe assays are also used to detect the presence of alpha−contamination. There is no other practical alternative to monitor for the presence of weak beta−emitters, especially tritium, than by swipe testing followed by liquid scintillation counting.
The Application Note on Swipe Assay Counting reviews the findings of several investigators who performed studies to evaluate the collection and counting efficiency of various swipe materials and methods for the detection of both beta− and alpha−radionuclide contamination. Studies indicate that pre-wetting the swipe with water or a 50:50 alcohol/water mixture improves the collection efficiency. Pre-wetting with dilute acid will probably provide the best collection efficiencies in swipe testing for alpha−contamination.
It is essential that the counting solution solubilizes both water-soluble and organic material. It is advisable to add a small amount of water to scintillation cocktails designed to accept water soluble samples; if the contaminant is water-soluble, the water solubilizes it from the surface of the solid support. Organic contaminants usually dissolve without any problem in an LSC cocktail.
The table below ranks, in order of performance, the overall efficiency of the various swipe media and wetting agents studied:
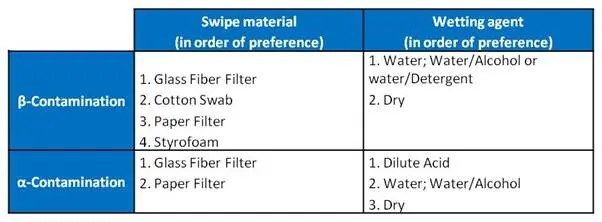
Swipe media
Swipe assays can also be performed on microplate readers.
Gels, TLC
The sample preparation method depends on the type of material on which the radiolabeled compound is collected. For example, agarose gels cannot be dissolved by the Soluene-350 solubilizer. However, when a section of agarose gel is placed in a 20 mL vial with 1 mL of sodium hypochlorite solution and incubated at 65°C for 45 minutes, it will completely dissolve.
The Application Note on Gel Counting presents several ways of preparing the sample matrix and combining it with the best-adapted scintillation cocktail to obtain the highest counting efficiency and most accurate results.
When the sample is eluted on thin layer chromatography plates, methods exist to recuperate the radiolabeled material to count the activity of each spot. The TLC plate is scraped, and the compound of interest is either eluted from the TLC-scrapings (by adding 1 mL of Soluene-350 followed by counting with Hionic-Fluor), or for water-soluble compounds the scrapings are resuspended in 1 mL of water with addition of a gelifying cocktail to prevent TLC particles from settling at the bottom of the vial.
CO2 counting
14C dating
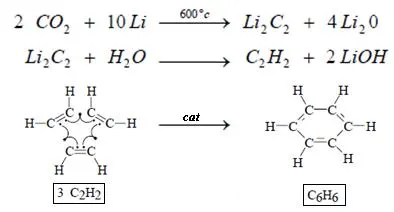
Carbon dating
Radiocarbon dating, or carbon dating, is a radiometric dating method that uses the naturally occurring radioisotope 14C to estimate the age of carbonaceous materials up to about 58,000 to 62,000 years. One of the most frequent uses of radiocarbon dating is to estimate the age of organic remains from archaeological sites. When plants fix atmospheric carbon dioxide (CO2) into organic material during photosynthesis, they incorporate a quantity of 14C that approximately matches the level of this isotope in the atmosphere (a small difference occurs because of isotope fractionation, but this is corrected after laboratory analysis). After plants die or are consumed by other organisms (for example, by humans or other animals) the 14C fraction of this organic material declines at a fixed exponential rate due to the radioactive decay of 14C. Comparing the remaining 14C fraction of a sample to that expected from atmospheric 14C allows the age of the sample to be estimated. The sample can be collected as radioactive CO2, and chemically transformed into benzene. Scintillators are then added for counting.
CO2(g) trapping
It is also possible to trap the gaseous CO2 released into alkaline solutions, forming carbonate, which stays in solution as long as the pH remains high enough. Several trapping agents exist and differ by quantity of CO2 that can be retained in the solution:
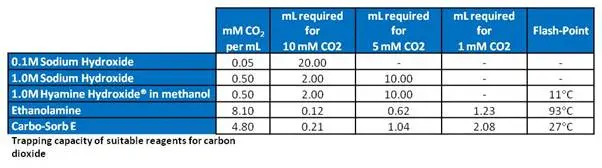
CO2 trapping
These trapping methods are commonly used for lab analyses such as the breath test.
Biofuel
Liquid scintillation counting of radiocarbon (14C) can also be used to determine the biological content of any organic material. All living biological material has a constant amount of radiocarbon per unit weight of total carbon, formed by cosmic radiation bombarding nitrogen molecules in the upper atmosphere. Radiocarbon will disappear from biological material through the radioactive decay process (half-life ~ 5730 years). Products fully made of fossil hydrocarbons do not include radiocarbons, as it has decayed completely within the millions of years the product has been underground. By determining the radiocarbon activity versus total carbon content, we can determine the biological content of any organic product.
Environmental samples
The advance of the nuclear industry in all its forms coupled with growing concerns of possible environmental contamination has led to an increased interest in the quantification of radioisotopes in the environment. Radionuclides currently present in the environment originate from a variety of sources, such as fallout from nuclear weapons testing, and in discharges from both nuclear and non-nuclear industries. Natural sources and fallout from nuclear devices provide the main input to terrestrial ecosystems, excepting certain radionuclides emanating from nuclear installations.
Because of both nuclear fallout and discharges from the nuclear industry, certain radionuclides are studied more than others. This is due to either their radiotoxicity, increased presence in the environment or ease of entry into the food chain.
There are three key application areas for LSC in environmental areas:
1. Measurement of natural series radionuclides
- Routine measurements in a range of sample matrixes (water, soil, air)
- Radon, Radium, Uranium, Thorium, etc.
2. Monitoring the environment around establishments associated with nuclear fuel cycle
- Beta emitters without significant gamma emissions (3H, 14C, 89Sr, 90Sr, 99Tc, 241Pu)
- These isotopes are monitored not only around nuclear installations, but also in the other end of the chain (3H in water and 14C in food)
3. Studying the rates of processes in the environment
- Radiocarbon dating
- Ground water movement and dating (3H)
- Marine productivity and particle flux
For research use only. Not for use in diagnostic procedures.
The information provided above is solely for informational and research purposes only. Revvity assumes no liability or responsibility for any injuries, losses, or damages resulting from the use or misuse of the provided information, and Revvity assumes no liability for any outcomes resulting from the use or misuse of any recommendations. The information is provided on an "as is" basis without warranties of any kind. Users are responsible for determining the suitability of any recommendations for the user’s particular research. Any recommendations provided by Revvity should not be considered a substitute for a user’s own professional judgment